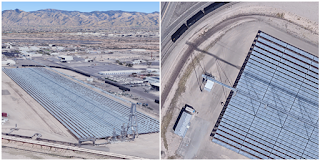 |
Fig. 1: The Sundt solar power plant in Tuscon, AZ |
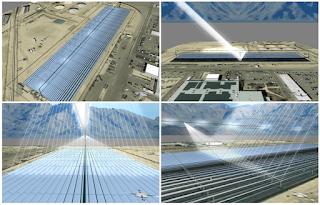 |
Fig. 2: Visualization of incident and reflecting light beams |
Tucson Electric Power (TEP) and AREVA Solar constructed a 5 MW compact linear Fresnel reflector (CLFR) solar steam generator at TEP’s H. Wilson Sundt Generating Station -- not far from the famous Pima Air and Space Museum. The land-efficient, cost-effective CLFR technology uses rows of flat mirrors to reflect sunlight onto a linear absorber tube, in which water flows through, mounted above the mirror field. The concentrated sunlight boils the water in the tube, generating high-pressure, superheated steam for the Sundt Generating Station. The Sundt CLFR array is relatively small, so I chose it as an example to demonstrate how
Energy3D can be used to design, simulate, and analyze this type of solar power plant. This article will show you how various analytic tools built in Energy3D can be used to understand a design principle and evaluate a design choice.
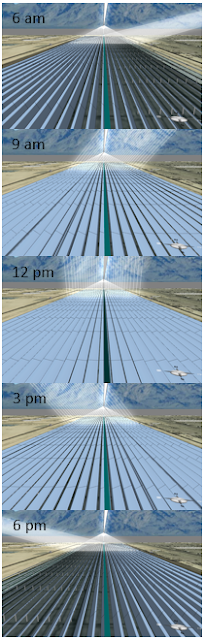 |
Fig. 3: Snapshots |
One of the "strange" things that I noticed from the Google Maps of the power station (the right image in Figure 1) is that the absorber tube stretches out a bit at the northern edge of the reflector assemblies, whereas it doesn't at the southern edge. The reason that the absorber tube was designed in such a way becomes evident when we turn on the light beam visualization in Energy3D (Figure 2). As the sun rays tend to come from the south in the northern hemisphere, the focal point on the absorber tube shifts towards the north. During most days of the year, the shift decreases when the sun rises from the east to the zenith position at noon and increases when the sun lowers as it sets to the west. This shift would have resulted in what I call the
edge losses if the absorber tube had not extended to the north to allow for the capture of some of the light energy bounced off the reflectors near the northern edge. This biased shift becomes less necessary for sites closer to the equator.
Energy3D has a way to "run the sun" for the selected day, creating a nice animation that shows exactly how the reflectors turn to bend the sun rays to the absorber pipe above them. Figure 3 shows five snapshots of the reflector array at 6am, 9am, 12pm, 3pm, and 6pm, respectively, on June 22 (the longest day of the year).
As we run the radiation simulation, the shadowing and blocking losses of the reflectors can be vividly visualized with the heat map (Figure 4). Unlike the heat maps for photovoltaic solar panels that show all the solar energy that hits them, the heat maps for reflectors show only the reflected portion (you can choose to show all the incident energy as well, but that is not the default).
There are several design parameters you can explore with Energy3D, such as the inter-row spacing between adjacent rows of reflectors. One of the key questions for CLFR design is: At what height should the absorber tube be installed? We can imagine that a taller absorber is more favorable as it reduces shadowing and blocking losses. The problem, however, is that, the taller the absorber is, the more it costs to build and maintain. It is probably also not very safe if it stands too tall without sufficient reinforcements. So let's do a simulation to get in the ballpark. Figure 5 shows the relationship between the daily output and the absorber height. As you can see, at six meters tall, the performance of the CLFR array is severely limited. As the absorber is elevated, the output increases but the relative gain decreases. Based on the graph, I would probably choose a value around 24 meters if I were the designer.
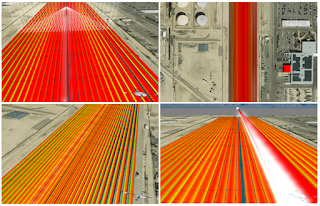 |
Fig. 4: Heat map visualization |
An interesting pattern to notice from Figure 5 is a plateau (even a slight dip) around noon in the case of 6, 12, and 18 meters, as opposed to the cases of 24 and 30 meters in which the output clearly peaks at noon. The disappearance of the plateau or dip in the middle of the output curve indicates that the output of the array is probably approaching the limit.
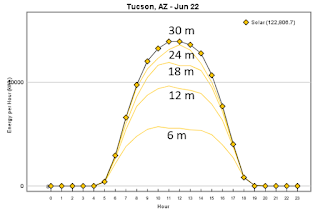 |
Fig. 5: Daily output vs. absorber height |
If the height of the absorber is constrained, another way to boost the
output is to increase the inter-row distance gradually as the row moves
away from the absorber position. But this will require more land.
Engineers are always confronted with this kind of trade-offs. Exactly
which solution is the optimal depends on comprehensive analysis of the
specific case. This level of analysis used to be a professional's job,
but with Energy3D, anyone can do it now.